Estimated reading time: 25 minutes
Get the original 1970 report that this blog post is based on here:
Table of contents
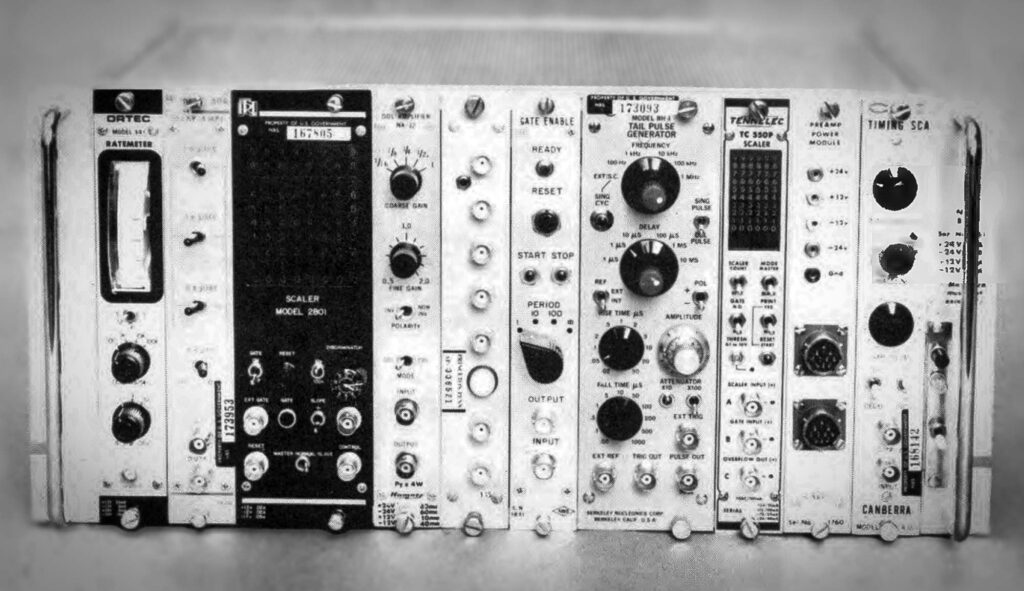
Overview
Nuclear Instrumentation Modules (NIM) play a key role in nuclear and particle physics research. They provide standardized, modular components for various applications. Developed in the 1960s, the NIM standard outlines mechanical dimensions, power supply voltages, and signal processing, ensuring compatibility and interchangeability among manufacturers.
NIM modules are used in fields like experimental particle physics, nuclear spectroscopy, radiation detection, medical physics, and environmental monitoring. These systems are valued for their modularity, flexibility, reliability, and high-performance analog processing. Additionally, they have limitations, such as limited digital communication, high power consumption, and the need for manual configuration.
Leading manufacturers like ORTEC, Canberra, Berkeley Nucleonics Corporation, FAST ComTec, and Stanford Research Systems continue to innovate within the NIM framework, which helps maintain the framework’s relevance in modern research.
Quality assurance is crucial in Nuclear Instrumentation Module production. Rigorous testing ensures reliability and performance. The NIM lexicon includes many specialized terms and components, reflecting the complexity and versatility of these systems.
There are about 50-100 different types of NIM modules commonly used. These are categorized into:
Miscellaneous utility modules
Signal processing
Logic and timing
Counting and analysis
Power supply
Specialized detector
Data acquisition
Pulse generation
Signal routing
Specialized analysis
Historical Development of NIM Standards
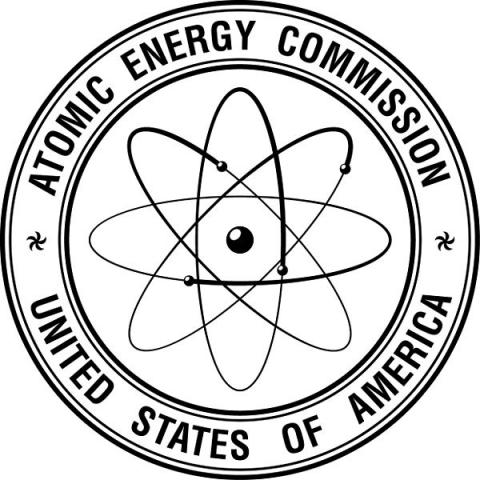
The development of NIM standards represents a significant milestone in the history of nuclear and particle physics instrumentation. Key milestones include:
- July 1964: The first NIM standard was established.
- November 1964: The first commercial Nuclear Instrumentation Module was introduced.
- 1968-1969: The U.S. Atomic Energy Commission published report TID-20893, formally defining the NIM standard.
- 1983: The NIM digital bus was introduced based on the IEEE 488 (GPIB) standard.
- 1990: The most recent revision of the Nuclear Instrumentation Module standard was published as DOE/ER-0457T.
The Nuclear Instrumentation Module standard’s success can be attributed to its simplicity, flexibility, and focus on analog signal processing. Furthermore, despite advances in digital instrumentation, NIM remains relevant in modern research environments.
NIM Applications
Nuclear Instrumentation Modules (NIM) are versatile and modular tools essential in many scientific and industrial applications. They are used in fields ranging from cutting-edge particle physics research to practical medical diagnostics and environmental monitoring.
NIM systems offer the precision, flexibility, and reliability needed to address complex challenges. In the following sections, we will explore how NIM modules are applied in various fields. We will highlight their critical roles and show how they contribute to advancements in technology and science.
Experimental Particle Physics
Nuclear Instrumentation Modules (NIM) are essential in experimental particle physics. They are widely used for signal processing, data acquisition, and timing measurements. In particle accelerators and collider experiments, NIM modules manage the detection and analysis of particles from high-energy collisions.
These modules are essential as they perform important tasks. They amplify weak signals from detectors, discriminate between different types of particles, and time the arrival of particles with high precision. NIM’s modular design enables researchers to create customized setups that can be easily reconfigured as experimental needs change.
The 2023 Particle Physics Project Prioritization Panel (P5) Report highlights recent advancements in particle physics. It emphasizes the need for ongoing support and innovation in experimental setups. NIM modules remain relevant in this context. They provide the necessary infrastructure for experiments exploring dark matter, dark energy, and other fundamental questions about the universe.
NIM systems are flexible and reliable. They are well-suited for both large-scale projects and smaller, specialized experiments. This ensures their continued utility in the field.
Nuclear Spectroscopy
Nuclear spectroscopy studies the energy spectra of nuclear radiation. NIM modules are crucial components in these setups. Common NIM modules include spectroscopy amplifiers, pulse shapers, and multi-channel analyzers (MCAs). They process and analyze signals from radiation detectors.
These modules help measure the energy and intensity of gamma rays, alpha particles, and other nuclear radiation. This provides valuable data for nuclear physics research and applications. In nuclear spectroscopy, the precision and stability of NIM modules are vital for high-quality data.
Modules like pulse-shape analyzers and single-channel analyzers (SCAs) allow researchers to distinguish between different types of radiation. They also help analyze complex spectra with high resolution. The ease of configuring and calibrating these modules makes NIM systems a preferred choice for both academic research and industrial applications in nuclear spectroscopy.
Radiation Detection
Radiation detection is a key application of NIM modules. They are used in nuclear power plants, medical facilities, and environmental monitoring stations. NIM modules like high-voltage power supplies, preamplifiers, and rate meters are essential in these systems. They provide the infrastructure needed to detect and measure ionizing radiation accurately.
These systems are crucial for ensuring safety and meeting regulatory standards in radiation environments. Advanced radiation detection systems often use NIM modules for real-time monitoring and data logging. For instance, neutron irradiation dose rate monitors and gamma area monitors depend on NIM components to measure radiation levels precisely.
NIM modules’ robust design and reliability make them suitable for continuous operation in demanding environments, ensuring the safety of both personnel and the public.
Medical Physics
Nuclear Instrumentation Modules (NIM) are crucial in medical physics, especially in diagnostic imaging and radiation therapy.
In diagnostic imaging, modules like pulse generators, amplifiers, and discriminators are used in systems such as positron emission tomography (PET) and single-photon emission computed tomography (SPECT). These systems need precise timing and signal processing to create high-resolution images and accurate dose measurements. As a result, NIM modules are essential for medical diagnostic equipment.
In radiation therapy, NIM modules ensure precise control of radiation doses, which is vital for effective treatment. Configuring and calibrating these systems allows for accurate delivery of radiation to target tissues while minimizing exposure to healthy tissues. The reliability and precision of NIM modules enhance the safety and effectiveness of treatments involving ionizing radiation.
Environmental Monitoring
Environmental monitoring involves detecting and measuring radiation levels to ensure public safety and regulatory compliance. NIM modules are crucial in monitoring stations. They measure background radiation, detect anomalies, and provide real-time data. Components like high-voltage power supplies, amplifiers, and rate meters ensure accurate and reliable measurements.
Portable NIM systems are also used for field measurements and emergency responses. These systems allow rapid deployment and real-time data collection in various environments. The flexibility and reliability of NIM modules make them ideal for environmental monitoring. They ensure accurate radiation assessments and prompt hazard identification.
Nuclear Safeguards
Nuclear safeguards involve monitoring and verifying nuclear materials to prevent their diversion for non-peaceful purposes.
NIM modules are essential in systems that detect and characterize nuclear materials. They provide critical data for safeguard measures. Modules such as pulse generators, amplifiers, and multi-channel analyzers (MCAs) are used to measure radiation signatures accurately.
The International Atomic Energy Agency (IAEA) and other regulatory bodies rely on advanced nuclear instrumentation, including NIM modules, to ensure treaty compliance.
The precision and reliability of NIM systems are vital for accurately detecting and characterizing nuclear materials. These systems support efforts to prevent nuclear proliferation and ensure global security.
Research Reactors
Research reactors serve various scientific and industrial purposes, including materials research, isotope production, and neutron scattering experiments.
NIM modules play a crucial role in research reactors’ instrumentation and control systems. They provide essential infrastructure for neutron flux monitoring, data acquisition, and signal processing. Researchers commonly use modules like amplifiers, discriminators, and time-to-digital converters (TDCs) in these setups.
NIM systems offer versatility, allowing researchers to configure and reconfigure experimental setups as needed. This flexibility supports a wide range of research activities.
The reliability and precision of NIM modules ensure accurate and consistent data collection. This accuracy enables researchers to conduct high-quality experiments and advance scientific knowledge in various fields.
Coincidence Measurements
Coincidence measurements detect two or more particles or radiation events simultaneously, revealing valuable information about their interactions.
Researchers use NIM modules like coincidence units, gate generators, and time-to-amplitude converters (TACs) for these measurements. These modules enable precise event correlation, which improves measurement accuracy.
In experiments involving radioactive decay, cosmic rays, or particle collisions, coincidence measurements identify events occurring simultaneously or within a specific time window. This technique is essential for studying rare events and understanding particle interactions.
NIM systems’ modularity and flexibility make them ideal for setting up and conducting coincidence measurements. These systems support a wide range of research activities.
Pulse Shape Analysis
Pulse shape analysis discriminates between different types of radiation by analyzing the shape of pulses produced in detectors.
Researchers use NIM modules like pulse shape analyzers and discriminators to process and analyze these pulses. This process allows them to distinguish between neutrons, gamma rays, and other radiation types. This technique proves particularly useful in experiments with multiple radiation types that need accurate identification.
Advanced pulse shape discrimination techniques, including those using machine learning, further enhance NIM systems’ capabilities. By precisely analyzing pulse shapes, researchers can improve measurement accuracy and gain deeper insights into various radiation types.
NIM modules’ flexibility and precision make them ideal for pulse shape analysis. These features support a wide range of research and industrial activities.
Education and Training
Educators widely use NIM modules to teach nuclear instrumentation techniques to students and trainees.
University laboratories and training centers offer hands-on experience with NIM systems. These systems help students learn signal processing, data acquisition, and experimental setup. The modular design of NIM systems lets students easily reconfigure modules for different experiments.
Training programs for nuclear technicians and engineers also use NIM modules to teach practical skills in instrumentation and radiation detection. Trainees gain valuable experience with NIM systems, preparing them for careers in nuclear research and related fields.
The widespread use of NIM modules in education ensures future scientists and engineers are well-prepared to advance nuclear technology.
Types of NIM Modules
Nuclear Instrumentation Module systems include a wide variety of specialized components.
While it’s difficult to provide an exact count, researchers commonly use approximately 50-100 distinct NIM modules.
These modules fall into several functional groups:
Signal Processing Modules
- Amplifiers (e.g., fast amplifiers, spectroscopy amplifiers)
- Discriminators (e.g., constant fraction discriminators, leading edge discriminators)
- Pulse shapers
- Delay modules
- Attenuators
- Linear gates
- Stretchers
Logic and Timing Modules
- Coincidence units
- Logic units (AND, OR, NOT gates)
- Gate and delay generators
- Time-to-Amplitude Converters (TAC)
- Time-to-Digital Converters (TDC)
Counting and Analysis Modules
- Scalers
- Rate meters
- Single Channel Analyzers (SCA)
- Multi-Channel Analyzers (MCA)
- Analog-to-Digital Converters (ADC)
Power Supply Modules
- High-voltage power supplies
- Low-voltage power supplies
Specialized Detector Modules
- Photomultiplier tube bases
- Preamplifiers for various detector types
Data Acquisition and Interface Modules
- CAMAC interfaces
- VME interfaces
- USB interfaces
Pulse Generation and Testing Modules
- Pulsers
- Test and calibration modules
Signal Routing and Distribution
- Fan-in/Fan-out modules
- Signal mixers
- Signal splitters
Specialized Analysis Modules
- Pile-up rejectors
- Spectrum stabilizers
- Peak sensing ADCs
Miscellaneous Utility Modules
- Level translators (e.g., NIM to TTL, ECL to NIM)
- Voltage-to-Frequency converters
- Current-to-Voltage converters
The modular nature of NIM systems allows researchers to combine these various module types to create complex, customized instrumentation setups tailored to specific experimental requirements.
NIM Advantages
Nuclear Instrumentation Modules (NIM) are indispensable in many scientific and industrial applications due to their versatility and modularity.
NIM systems offer precision, flexibility, and reliability, essential for tackling complex challenges. Researchers use them in cutting-edge particle physics, medical diagnostics, and environmental monitoring.
Several significant advantages explain the widespread adoption and lasting relevance of NIM systems across different fields. These benefits make NIM systems the preferred choice for researchers and technicians needing reliable, flexible instrumentation.
Let’s examine the key advantages of NIM modules, focusing on how their design and functionality enhance their effectiveness and popularity.
Modularity and Flexibility
NIM systems offer significant advantages, particularly in their modularity and flexibility.
Thanks to their standardized dimensions and electrical specifications, researchers can easily mix and match NIM modules. This modular approach allows them to customize instrumentation setups efficiently. Swapping out or upgrading individual modules without redesigning the entire system provides valuable flexibility.
This flexibility is crucial in research environments where experimental requirements change rapidly. In particle physics experiments, researchers can quickly reconfigure NIM modules to adapt to new hypotheses or conditions. This adaptability reduces downtime and keeps experiments on track.
The modular design of NIM systems also supports incremental upgrades. Laboratories can update equipment with the latest technology without the cost of complete system overhauls.
Standardization
The Nuclear Instrumentation Module (NIM) standard ensures that modules from different manufacturers remain compatible and interchangeable.
This standardization simplifies component selection and integration, allowing researchers to confidently combine modules from various sources. The NIM standard specifies power supply voltages, signal levels, and mechanical dimensions, providing a reliable framework for designing complex systems.
Standardization also promotes collaboration and knowledge sharing within the scientific community. Researchers can easily replicate and build upon each other’s work, knowing their NIM-based setups will remain compatible.
This interoperability fosters innovation and accelerates scientific progress, allowing new discoveries and techniques to spread quickly across laboratories and institutions.
Reliability
NIM modules offer robust construction and reliable performance.
Leading manufacturers employ stringent quality control processes to ensure each module meets high standards for accuracy and durability. This reliability is crucial in experimental setups where consistent performance ensures accurate and reproducible results.
In demanding environments like nuclear power plants or high-energy physics experiments, NIM modules provide researchers and technicians with peace of mind. Their robust design withstands continuous operation, reducing equipment failures and minimizing downtime.
This reliability makes NIM systems indispensable in critical applications where precision and consistency are essential.
Ease of Maintenance
The modular design of NIM systems simplifies maintenance and troubleshooting.
When a module fails or needs calibration, it can be easily removed and replaced without disrupting the system. This ease of maintenance reduces downtime and quickly restores experimental setups to full functionality.
The widespread availability of NIM modules ensures that replacement parts are readily accessible. Researchers and technicians can source new or refurbished modules from various suppliers, keeping their systems operational even as components age.
The straightforward maintenance of NIM systems enhances their long-term viability and cost-effectiveness.
Wide Range of Functions
NIM modules offer a diverse array of functions, including signal amplification, pulse shaping, timing, logic operations, and data acquisition.
This broad functionality enables researchers to build complex systems tailored to their specific experimental needs. Whether you conduct particle physics experiments, nuclear spectroscopy, or environmental radiation monitoring, NIM modules can meet your requirements.
Specialized modules allow researchers to achieve high precision and performance in their measurements. For example, pulse shape analyzers and constant fraction discriminators provide precise timing and energy resolution in nuclear spectroscopy.
NIM systems’ versatility ensures adaptability across various scientific and industrial applications, making them invaluable tools for researchers.
High-Performance Analog Processing
NIM modules excel in analog signal processing, especially in applications requiring high precision and low noise.
The analog nature of NIM modules allows researchers to accurately amplify, shape, and analyze signals from radiation detectors. This high-performance processing is crucial in applications like nuclear spectroscopy, where precise energy measurement is critical.
Advanced techniques such as pulse shaping and discrimination enable researchers to extract detailed information from detector signals. By processing analog signals with high fidelity, NIM systems ensure accurate and reliable data, supporting quality research and experimentation.
This emphasis on analog performance sets NIM modules apart from many modern digital systems, making them indispensable in applications where analog precision is essential.
Educational Value
The modular and visual design of Nuclear Instrumentation Module (NIM) systems makes them ideal for teaching nuclear instrumentation techniques.
University laboratories and training centers use NIM modules to provide hands-on experience in signal processing, data acquisition, and experimental setup. Students can easily configure and reconfigure NIM systems, gaining practical insights into nuclear instrumentation principles.
NIM modules are also a part of nuclear technician and engineer training programs. By working with these systems, trainees develop the skills needed to operate and maintain nuclear instrumentation in real-world settings.
NIM systems’ educational value ensures that the next generation of scientists and engineers is well-equipped to advance nuclear technology and contribute to ongoing research.
Longevity and Backwards Compatibility
The NIM standard, developed in the 1960s, remains relevant due to its well-conceived design and adaptability.
Manufacturers often ensure new NIM modules are backward compatible with older systems, allowing for gradual upgrades and long-term use. This longevity ensures NIM systems continue to provide value over extended periods.
Backward compatibility also allows researchers to integrate new technologies with existing setups. Researchers can add advanced modules to their systems without replacing all existing equipment, ensuring a smooth transition to newer capabilities.
This adaptability has maintained NIM systems’ enduring popularity in research and industrial applications.
Specialized Power Distribution
The NIM bin delivers a standardized power distribution system optimized for nuclear instrumentation, including both positive and negative voltages.
This reliable power supply ensures that modules operate within their specified parameters, maintaining accurate and stable measurements.
Precise voltage and current control are critical in applications requiring specialized power distribution. For example, high-voltage power supplies are essential for operating photomultiplier tubes and other radiation detectors.
By providing stable and accurate power, NIM systems deliver consistent performance, supporting high-quality data acquisition and analysis.
Fast Logic Capabilities
The NIM logic standard, with its current-based signaling, enables high-speed logic operations.
This capability proves especially useful in timing-critical applications like coincidence measurements, where precise timing is crucial for event correlation. Fast logic allows researchers to detect and analyze events with high temporal resolution, improving measurement accuracy.
In high-energy particle collisions, fast logic modules like constant fraction discriminators and time-to-digital converters (TDCs) are crucial for capturing rapid events. This supports advanced research in nuclear and particle physics.
NIM Limitations
Nuclear Instrumentation Modules (NIM) offer many advantages, but they also present certain limitations.
These limitations can impact their applicability in modern research environments. Researchers and technicians need to understand these challenges to make informed decisions about their instrumentation needs.
In this section, we explore the key limitations of NIM systems and highlight the challenges and constraints associated with their use.
Limited Digital Communication
NIM systems face a primary limitation in their limited digital communication capabilities.
Unlike modern standards like CAMAC or VMEbus, NIM modules don’t communicate through the crate backplane. This lack of a standardized digital bus restricts integrating complex digital data acquisition and control systems within an NIM framework.
This limitation proves challenging in experiments requiring high-speed data transfer and complex triggering schemes. Researchers often need additional interfaces or hybrid systems to incorporate digital communication, adding complexity and potential failure points.
As digital instrumentation advances, NIM systems’ limited communication may increasingly constrain their applicability in certain research areas.
Power Consumption
NIM modules, especially older designs, can consume significant power.
Using discrete components or early integrated circuits often leads to increased power consumption. This higher consumption generates more heat, requiring additional cooling and robust power supplies to ensure stable operation. In large experimental setups, where multiple NIM modules operate simultaneously, this can become a significant concern.
High power consumption may pose a drawback in environments where power efficiency is crucial. Researchers must balance NIM’s analog processing benefits with the need for efficient power management. They may explore alternative solutions or newer module designs offering better power efficiency.
Size and Density
The standardized size of NIM modules, while consistent, can be relatively large compared to modern, compact electronics.
This larger size can limit instrumentation density in space-constrained environments, making it challenging to fit all necessary components within a limited footprint. The bulkiness of NIM modules may also affect the portability of instrumentation setups.
Researchers in small laboratories or field environments might find these size and density limitations restrictive. They may need to consider alternative solutions. Although NIM’s modularity offers flexibility, the physical constraints of the modules can impact the design and implementation of experimental setups.
Cost
High-quality NIM modules, especially for specialized applications, can be expensive.
The cost per function often exceeds that of more integrated modern systems, making NIM systems a significant investment for research institutions. Using multiple modules to achieve specific functionalities can further increase the overall cost of a NIM-based setup.
Budget constraints may limit research groups from adopting NIM modules, despite their long-term reliability and performance. Researchers need to assess the cost-benefit ratio relative to their needs and seek funding sources for acquisition.
Limited Data Throughput
NIM systems lack a standardized high-speed digital bus, limiting their data throughput capabilities.
This limitation poses a significant challenge in experiments requiring rapid transfer and processing of large data volumes. While NIM modules excel in analog signal processing, their limited throughput hinders their use in high-speed digital data acquisition.
Researchers often need to supplement NIM systems with additional digital hardware or hybrid setups. This approach increases complexity and demands extra expertise to integrate and manage different components effectively.
Analog Nature
NIM modules excel in analog signal processing but can fall short in increasingly digital research environments.
Modern experiments often require seamless integration between analog and digital systems for advanced data acquisition. The analog focus of NIM modules may require additional interfaces and conversion steps to bridge this gap.
These extra steps can introduce errors and complexity in data workflows. Researchers must carefully design setups to ensure accurate and efficient integration. By addressing these digital limitations, they can fully leverage the strengths of NIM modules.
Lack of Built-in Processing
NIM modules lack built-in microprocessors or FPGAs for advanced signal processing and decision-making.
This absence limits their ability to perform complex data analysis and real-time processing within the NIM framework. Researchers often rely on external processing units or hybrid systems to achieve the desired functionality.
Without built-in processing, NIM systems demand more manual configuration and calibration, adding complexity to experimental setups. While NIM’s modularity offers flexibility, the need for extra processing capabilities complicates the design and implementation of instrumentation systems.
Manual Configuration
NIM modules often require manual configuration via front-panel controls, which can be time-consuming and error-prone.
This manual process complicates achieving precise, consistent settings across complex setups with multiple modules. Replicating setups accurately becomes more challenging, impacting workflow efficiency.
Researchers must invest extra time in calibrating and configuring NIM systems, potentially slowing down their work. While hands-on configuration benefits education, it limits NIM’s usefulness in high-throughput or automated environments where rapid, consistent setups are essential.
Limited Scalability
The physical size of NIM crates and power distribution limits scalability in large experiments.
As module numbers increase, power demands and space constraints become significant challenges, hindering effective scaling of experimental setups.
Researchers must carefully plan instrumentation layouts and power distribution to accommodate larger setups. They may need to explore alternative or supplementary systems to achieve the necessary scalability.
While NIM systems offer flexibility and modularity, their scalability limitations can impact large-scale research projects.
Obsolescence of Some Components
As electronics advance, older NIM module components may become obsolete, complicating repairs and replacements.
Diminishing part availability can threaten the long-term viability of existing NIM systems. Researchers may need to source replacements from secondary markets or upgrade to newer modules.
Although NIM modules offer backward compatibility, component obsolescence remains a concern. Researchers must weigh the benefits of maintaining existing systems against the need for periodic upgrades to ensure continued reliability.
Leading NIM Manufacturers
Several key manufacturers have driven the development and success of NIM systems.
These companies have a rich history of innovation and continue to lead in nuclear instrumentation. They provide high-quality NIM modules and related equipment, remaining pivotal in the industry.
Below, we highlight some leading NIM manufacturers, detailing their contributions and current industry standing.
ORTEC
ORTEC, originally Oak Ridge Technical Enterprise Corporation, pioneered the NIM market.
Founded in 1960, ORTEC quickly became a major nuclear instrumentation supplier, offering 17 NIM modules in its 1966 catalog. Proximity to major national labs, like those run by the Atomic Energy Commission, fueled its early success. Now part of PerkinElmer, ORTEC continues providing a wide range of NIM modules and nuclear measurement solutions.
ORTEC is known for its comprehensive suite of NIM modules, including amplifiers, discriminators, and pulse generators, vital for nuclear and particle physics experiments. The company’s commitment to quality and innovation keeps its products reliable in modern research. Today, ORTEC remains a leading provider of nuclear instrumentation, supporting scientific advancements and industrial applications globally.
Canberra
Emery Olcott and Chuck Greer founded Canberra Industries in 1965, quickly becoming a leader in the NIM market.
By 1976, Canberra offered around 100 NIM modules, addressing a broad range of nuclear measurement needs. Strategic acquisitions, including Packard Instruments, further strengthened Canberra’s industry position. The company advanced nuclear instrumentation with innovations in gamma spectroscopy, radiation monitoring, and environmental assessment.
Canberra’s product line has since evolved to include cutting-edge digital systems and software, complementing its traditional NIM offerings. Known for quality and strong customer support, Canberra has earned a reputation as a trusted partner in nuclear research and industry. Today, Canberra continues to innovate, expanding its product portfolio to meet the needs of modern nuclear science and technology.
Berkeley Nucleonics Corporation (BNC)
Berkeley Nucleonics Corporation (BNC), founded in 1963, quickly became a leading manufacturer of precision electronic instrumentation.
Initially known for custom pulse generators, BNC expanded its range to include digital delay generators, RF/microwave signal generators, and nuclear instrumentation modules. BNC’s commitment to precision and reliability has earned industry-wide respect.
BNC specializes in pulse generators, delays, and other crucial NIM modules for nuclear and particle physics experiments. The company’s innovative designs and focus on quality ensure its products meet modern research demands. BNC continues to support the scientific community with advanced instrumentation, maintaining a strong presence in both domestic and international markets.
FAST ComTec
FAST ComTec, a leading manufacturer of NIM bins and power supplies, has earned a reputation for reliability and quality.
Researchers widely use FAST ComTec’s NIM bins for their robust construction and long-term reliability in nuclear and particle physics. The company’s power supplies deliver the voltages and stability needed for precise measurements.
Beyond NIM bins and power supplies, FAST ComTec offers specialized modules for data acquisition and signal processing. By focusing on innovation and quality, FAST ComTec ensures its products remain effective in modern research.
FAST ComTec continues to support the scientific community with advanced instrumentation, addressing the evolving needs of nuclear and particle physics research.
Stanford Research Systems (SRS)
Founded in 1980, Stanford Research Systems (SRS) produces high-quality scientific and engineering instruments.
SRS is known for its test and measurement instruments, including analog PID controllers, audio analyzers, programmable temperature controllers, and digital delay generators. These products deliver precise, reliable measurements across various fields, including nuclear instrumentation.
SRS’s NIM modules offer advanced analyzers and specialized capabilities, making them essential for nuclear and particle physics researchers. By committing to innovation and quality, SRS ensures its products meet the rigorous demands of modern research.
SRS remains a leading provider of scientific instrumentation, driving advancements in technology and science across various disciplines.
Tennelec
Founded in the 1960s, Tennelec (originally Tennessee Electronics) quickly became a key supplier of NIM modules.
Tennelec gained recognition for its high-quality linear amplifiers, scalers, and rate meters, widely used in nuclear research. These products significantly advanced radiation detection and measurement in laboratories.
In the 1990s, Oxford Instruments acquired Tennelec, which later became part of Canberra Industries. Despite these ownership changes, Tennelec’s legacy of innovation and quality continues to influence the industry.
Today, Tennelec’s products remain reliable and high-performing, holding a valued place in Canberra’s extensive portfolio.
Other Significant Companies
In addition to the major players, several smaller companies have made significant contributions to the NIM market. LeCroy (LRS) is known for producing both NIM and CAMAC modules, specializing in fast electronics for high-energy physics experiments. Nuclear Data (ND), another notable company, was later acquired by Canberra but made important advancements in data acquisition and analysis modules.
Other companies, such as Tracor-Northern, Metronics, and Victoreen/Tullamore, have also contributed to the development and production of NIM modules. These smaller companies have contributed specialized products and innovations that have enriched the NIM ecosystem. Their efforts have ensured a diverse range of options for researchers and supported the continued evolution of nuclear instrumentation technology.
Conclusion
The Nuclear Instrumentation Module (NIM) standard has proven reliable and flexible for nuclear and particle physics research.
Its modular design and standardized specifications allow researchers to create customized, high-performance setups tailored to specific needs. Despite newer integrated digital systems, NIM remains invaluable, especially in applications requiring precise analog signal processing.
As technology evolves, the NIM standard will likely adapt, incorporating advancements while maintaining modularity and standardization. Ongoing efforts from leading manufacturers and the development of new modules ensure NIM’s continued relevance.
Understanding NIM is essential for effective experimentation and data acquisition in nuclear and particle physics. This guide provides valuable insights for researchers and technicians seeking to deepen their knowledge of NIM systems and applications.
NIM Lexicon/Glossary/Terminology
General Terms
- NIM Bin: The power supply and housing unit for NIM modules.
- NIM Logic: A current-based logic standard used in NIM systems.
- Single-width Module: The standard size for a NIM module, measuring 1.35 inches (34 mm) wide.
- Double-width Module: A NIM module that is twice the width of a standard module.
- Faceplate: The front panel of a NIM module, typically containing controls, inputs, and outputs.
- Backplane: The rear part of the NIM bin that provides power and sometimes signal connections to the modules.
- BNC Connector: A type of RF connector commonly used for signal input and output.
- LEMO Connector: Another type of connector used in NIM systems, often for higher-density modules.
- Fast NIM: Refers to NIM modules designed for high-speed timing applications.
- Slow NIM: Refers to NIM modules used for slower, more precise measurements like spectroscopy.
- ADC (Analog-to-Digital Converter): A module used to convert analog signals to digital form.
- TDC (Time-to-Digital Converter): Used for precise timing measurements in NIM systems.
- Discriminator: A module that produces a logic pulse when an input signal exceeds a set threshold.
- Coincidence Unit: A module that detects when multiple input signals occur simultaneously or within a specified time window.
- Scaler: A module used for counting pulses or events in NIM systems.
- Gate Generator: A module that produces logic pulses of specified width and delay.
- Fan-in/Fan-out: Modules used to combine multiple input signals or distribute a single signal to multiple outputs.
- Delay Module: Used to introduce precise time delays in signal paths.
- Pulse Shaper: A module that modifies the shape of input pulses for optimal processing.
- ECL Logic: Emitter-Coupled Logic, an alternative logic standard sometimes used in NIM systems for high-speed applications.
Specialized Terms
- CFD (Constant Fraction Discriminator): Generates timing signals independent of pulse amplitude variations.
- TAC (Time-to-Amplitude Converter): Converts the time interval between two pulses into an analog voltage pulse.
- SCA (Single Channel Analyzer): Produces a logic output when the input pulse amplitude falls within a specified range.
- MCA (Multi-Channel Analyzer): Sorts and counts input pulses according to pulse height.
- Pile-up Rejector: Detects and rejects overlapping pulses.
- Linear Gate: Allows or blocks the passage of analog signals based on a logic control input.
- Stretcher: Extends the duration of short pulses for easier measurement by slower ADCs.
- Rate Meter: Converts pulse frequency to an analog voltage.
- Mixer: Combines multiple analog signals.
- Attenuator: Reduces signal amplitude by a fixed or variable amount.
- FIFO (First-In-First-Out) Buffer: Used for temporary data storage in high-speed data acquisition systems.
- CAMAC (Computer Automated Measurement And Control): A modular data handling system often used alongside NIM for digital data acquisition.
- VME (Versa Module Europa): Another modular standard for data acquisition and control, sometimes used in conjunction with NIM systems.
- NIM-ECL Translator: Converts between NIM logic levels and ECL (Emitter-Coupled Logic) levels.
- Octal Discriminator: A discriminator module with eight independent channels in a single-width NIM module.
- Pulser: Generates precise electrical pulses for testing, calibrating, and simulating detector signals.
- MHV (Medium High Voltage): A coaxial connector designed for medium-high voltage applications, typically rated for 2-3 kV in DC service.
- SHV (Safe High Voltage): A specialized coaxial connector designed for high voltage applications, rated for up to 5000 volts DC and 5 amperes.
NIM Specifications
Mechanical Specifications
- Standard module width: 1.35 inches (34 mm)
- Maximum faceplate height: 8.7 inches (221 mm)
- Maximum module depth: 9.7 inches (246 mm)
- Modules can be built in multiples of the standard width (double-width, triple-width, etc.)
Electrical Specifications
- Power supply voltages: ±12V and ±24V DC (mandatory)
- Optional power supply voltages: ±6V DC and 110V or 220V AC
- NIM logic standard: Current-based logic with negative “true” at -16 mA into 50 ohms (-0.8 volts) and 0 mA for “false”
- An ECL-based logic option is also specified
Crate (NIM bin) Specifications
- Provides power to modules via a backplane connector
- Standard pin assignments for power and signal distribution
Cabling and Connectors
- BNC connectors are commonly used for analog and logic signals
- LEMO connectors may be used for higher density modules
- RG-58 (50 ohm) coaxial cable is typically used for timing and logic signals
- RG-62 (93 ohm) coaxial cable is often used for spectroscopy signals